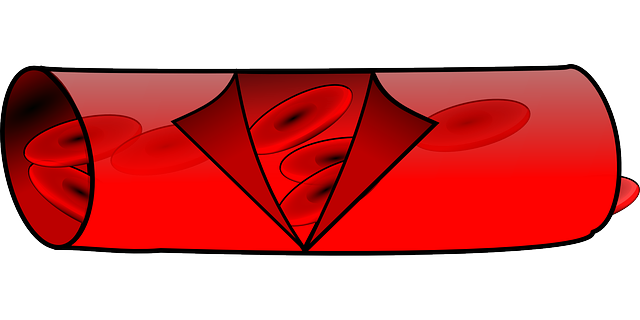
A New Biomaterial for Medical 3D Printing
Graphene oxide is a substance known for its flexibility. It’s true in a literal sense, as when it is used in solar cells or electronics, and true in the sense that it is extremely versatile.
In fact, graphene oxide — i.e., an oxidized form of graphene — can be found in such divergent items as lithium batteries, sensors and membranes. And in March 2020, an international team of scientists discovered that when that substance was 3D-printed in combination with a certain protein, it could form what were described as “tissue-like vascular structures.”
This represents the latest advance in the development of artificial blood vessels, which in time could be used to treat everything from cardiovascular disease to gunshot wounds.
The study, headed by Professor Alvaro Mata at the University of Nottingham/Queen Mary University London, concluded that the graphene oxide and protein went through a process of self-assembly, defined as the process through which various components interact to form new, functional structures. More specifically, the graphene oxide provides the framework for what Mata described in a news released as “micro-scale capillary-like fluidic structures that are compatible with cells, exhibit physiologically relevant properties, and have the capacity to withstand flow.”
Dr. Yuanhao Wu, the project’s lead researcher, noted in that same release that self-assembly on a molecular level had until now been “limited” despite the scientific community’s best efforts. This breakthrough, she added, represents a technique that “can be easily integrated with additive manufacturing to easily fabricate biofluidic devices that allow us (to) replicate key parts of human tissues and organs in the lab.”
That could have particular implications for patients undergoing bypass surgery as a result of cardiovascular disease, which according to the World Health Organization is the number one cause of death around the globe.
Research in the area of artificial blood vessels has, as a result, taken on added weight, and dates back to 1986, when scientists attempted to make them from bovine aortic cells. Particularly promising were two discoveries in 2017 — one in China, and one in the United States, at the University of California San Diego — through the use of 3D printers.
The Chinese researchers combined stem cells and nutrients to construct a graft that was used to replace a portion of an artery in the abdomens of 30 rhesus monkeys, while the U.S. scientists created a graft — subsequently used in mice — out of vascular cells that were converted into hydrogels.
Then, in 2019, researchers from Yale University and a Durham, N.C.-based medical technology firm called Humacyte used arterial cells from cadavers to create a collagen/protein structure known as a cellular matrix — i.e., the scaffolding that gives blood vessels their shape. They were then implanted in the arms of patients suffering from kidney disease, and over time came to be populated by the patients’ own cells.
The discovery in March of this year represents the next step in the creation of artificial blood vessels, and may someday prove to be a giant leap forward. Given the prevalence of cardiovascular disease, it is impossible to overstate the importance of such work, and what it might mean in the future.
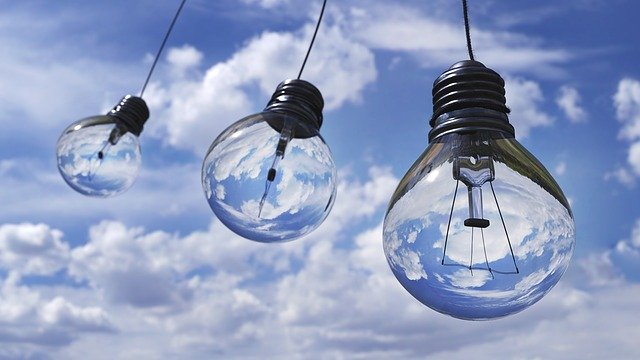
How Graphene Could Replace Mercury in Lighting
Over the past 20 years, there has been a strong push to develop more efficient solutions for artificial lighting. Incandescent bulbs were the dominant technology for over 100 years, but by the 2000s, environmentalists concerned with the limited energy efficiency and waste generated by incandescent bulbs started promoting longer-lasting CFL bulbs, as well as LED lights. Lasting years longer than traditional incandescent bulbs, CFL and LED lighting generate much less waste over the same amount of time.
But there remains one major concern: They contain mercury, which poses a potentially significant health hazard if the bulbs are not safely discarded.
Enter graphene. A company based in Manchester, England, called Graphene Lighting first produced lightbulbs featuring LED filaments coated in graphene in 2015. The bulbs, which contain no mercury, are 10 percent more energy-efficient than LED lights, owing to graphene’s superior conductivity. Besides obviously being more environmentally friendly than their predecessors, the bulbs are also more durable, lasting 25,000 hours, and are more cost-effective.
Graphene was first developed by two Russian scientists, Andre Geim and Konstantin Novoselov, at the University of Manchester in 2004. The school’s deputy vice chancellor, Professor Colin Bailey, is one of the directors of Graphene Lighting, which was founded in 2014. The Canadian-financed company added a second plant, in Shenzhen, China, five years later.
That same year brought another graphene-related lighting development, as researchers at the Norwegian University of Science and Technology created ultraviolet light on a graphene surface for the first time. One of the researchers, Ida Marie Hoiaas, told Phys.org that such a product “could turn out to be cheaper and more stable and durable than today’s fluorescent lamps.”
“If we succeed in making the diodes efficient and much cheaper,” Hoiaas told Phys.org, “it’s easy to imagine this equipment becoming commonplace in people’s homes. That would increase the market potential considerably.”
According to that same outlet, the process began in a Japanese laboratory, where a layer of graphene was placed on glass. Through a process known as molecular beam epitaxy, nanowires composed of aluminum gallium nitride were grown on the graphene substrate, and the sample was shipped off to the researchers in Norway.
They constructed gold and nickel contacts on the graphene and nanowires. The graphene then served as the conductor when power was sent to the nanowires, which emitted the ultraviolet light. Graphene is transparent to all light, regardless of wavelength, so that which was produced in this case shone through the graphene, as well as the glass.
Not only is graphene a more cost-effective alternative to LEDs and CFL bulbs, it is also non-toxic, since it does not use mercury. Use of that element in fluorescent lighting has come under criticism from various organizations, including the UN, leaving industries scrambling to find new alternatives in order to meet new health and safety regulations.
Graphene’s potential, evident in so many other areas, has, shall we say, been brought to light once again. The use of this nanomaterial makes for lighting options that are cheaper, safer and more efficient than those that came before, and very well might serve as a beacon to the future.
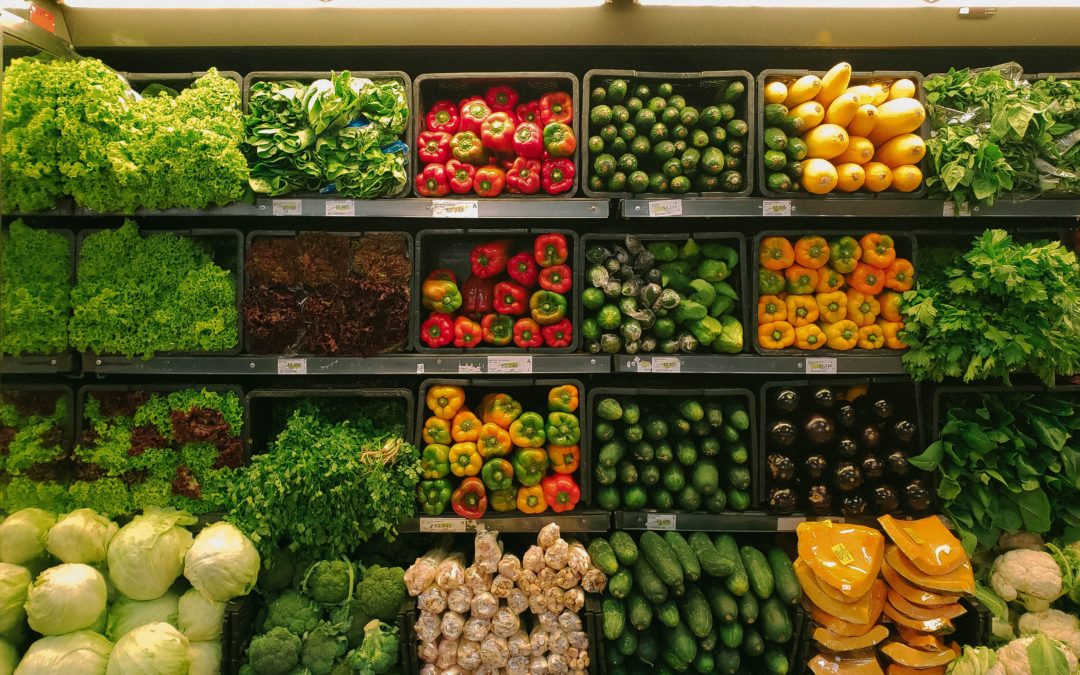
How Nanomaterials Are Extending Food Shelf Life
Most of us can identify with the feeling of purchasing food at the grocery store and forgetting to eat it until it was too late. Unfortunately, forgetting food leads to an enormous amount of food waste, creating higher costs for companies, consumers and the environment.
This issue could now be alleviated, thanks to a company named NanoPack. This company is experimenting with food-safe film that will extend the shelf life of foods through the use of nanomaterials, leading to higher convenience and savings for the consumer and producers alike.
In tests, NanoPack found that their film could extend the shelf life of bread for three weeks. It also showed that the lives of cherries and yellow cheese could be extended by up to 40 percent. This additional time on shelf could offer huge savings to consumers who may not anticipate life events getting in the way of their cooking. It benefits single individuals who may not be able to consume large quantities of food before it spoils. Extending a food’s shelf life also reduces the waste that companies must produce in order to get the food on the shelves in the first place.
NanoPack’s innovative food film works by using both antimicrobial nanomaterials and essential oils. The combination of these two elements aids in slowing the development of bacteria on food which leads to rotting and decay. The essential oils used are developed from oregano and thyme, making it safe for consumption. This is important, as leery consumers may not be excited about consuming experimental food preservation materials. NanoPack ensures that all of their films are safe for all.
While the film has shown initial promise extending the shelf life of bread, cheese, and cherries, NanoPack has high hopes that their film will prove efficacious on many other foods too. Such an advance across the industry might decrease the need for our reliance on preservatives. And while preservatives hold an important place in food production today, their removal would mean fewer problems for the more sensitive and allergenic among us.
NanoPack says that it will take at least another year or two to fully develop its shelf-life-increasing film. However, they are already testing it on other foods, and the product shows quite great promise for consumers and producers alike.
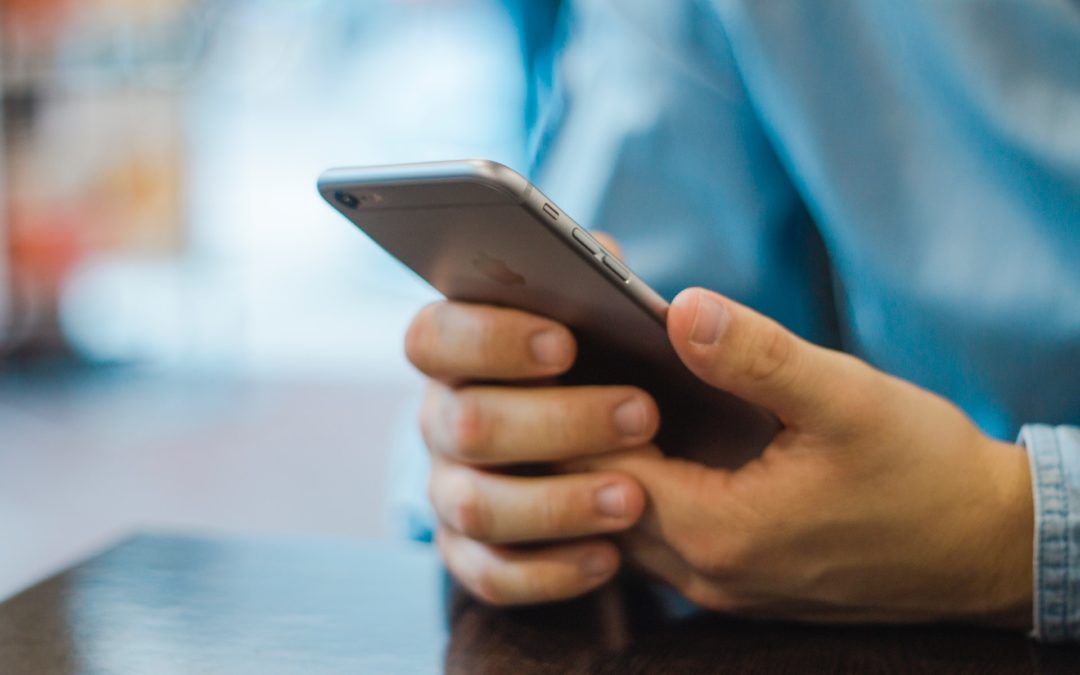
Graphene For Smartphone Applications
For anyone not familiar with the highly advanced material known as graphene, it is the first two-dimensional substance that mankind has ever had the pleasure of working with. As anyone with a physics background can point out, most earthly materials have a 3D structure. Unlike these more conventional materials, graphene is comprised of only one layer of carbon-based atoms. In a nutshell, graphene is a single sheet made out of carbon and that sheet has the overall thickness of one solitary atom.
The material known as graphene is dramatically changing the smartphone world, as it has technological applications capable of revolutionizing not just smartphones, but the greater computer industry as a whole. Graphene is capable of being used for improved CPUs, longer-lasting batteries, foldable or flexible display screens, and more. Here are some of the ways that graphene can be used to improve smartphones.
- Foldable and Flexible Display Screens
Leading smartphone brands are already offering devices that possess display screens that can be folded or are extremely flexible. While these screens are made out of thin and flexible sheets of highly specialized plastic, it is widely thought that this plastic material will eventually be replaced by graphene. Kostya Novoselov, one of two people that discovered graphene, is currently working on a 2D LED screen prototype that uses metallic graphene and LEDs at the atomic level.
- Silicon Chip Replacement
The standard silicon chips are created in such a way to reduce heat accumulation and increase conductivity within computer processing systems. Several isolated studies have shown that silicon chips made out of graphene are able to achieve a super-conductive ability while reducing core operating temperatures by more than 55 degrees Fahrenheit or 13 degrees Celsius. While these graphene chips are still in the early stages of development, they are likely to be extremely revolutionizing in how they help computer technology evolve.
- Supercapacitor Batteries
Most batteries in this day and age contain electrochemical power cells such as lithium-ion. The next-generation smartphone batteries are likely to have supercapacitors abilities, which means that they will store energy within an isolated electrical field, rather than relying on a controlled reaction of mixing chemicals. Due to the fact that industrially synthesized graphene will be far cheaper than modern battery components, it will be much cheaper for battery manufacturers to create supercapacitor units which will greatly benefit smartphone consumers.
- Better Mobile Camera Sensors
A college professor at Nanyang Technological University in Singapore named Wang Qijie has become somewhat famous for recently inventing an extremely cheap and highly flexible photo sensor made entirely out of graphene. The graphene-based sensor is 1,000 times more sensitive to light than any of the run-of-the-mill camera sensors being used today. What is even more interesting is that graphene camera sensors use far less energy and they will be substantially cheaper to produce on an industrial manufacturing level.
- Faster Mobile-based Internet
A highly celebrated group of researchers from the universities of Bath and Exeter have recently proven that graphene used in optical switches would be able to deliver the internet 100 times faster than all currently available telecommunications infrastructure. The optical response rate of graphene is super high and if improved upon beyond what the researchers were able to model, it could eventually deliver internet speeds that are nearly unfathomable when considering the speeds that are available today. Several leading telecommunication companies are already exploring ways to incorporate test trials of graphene made components into their infrastructural networks.
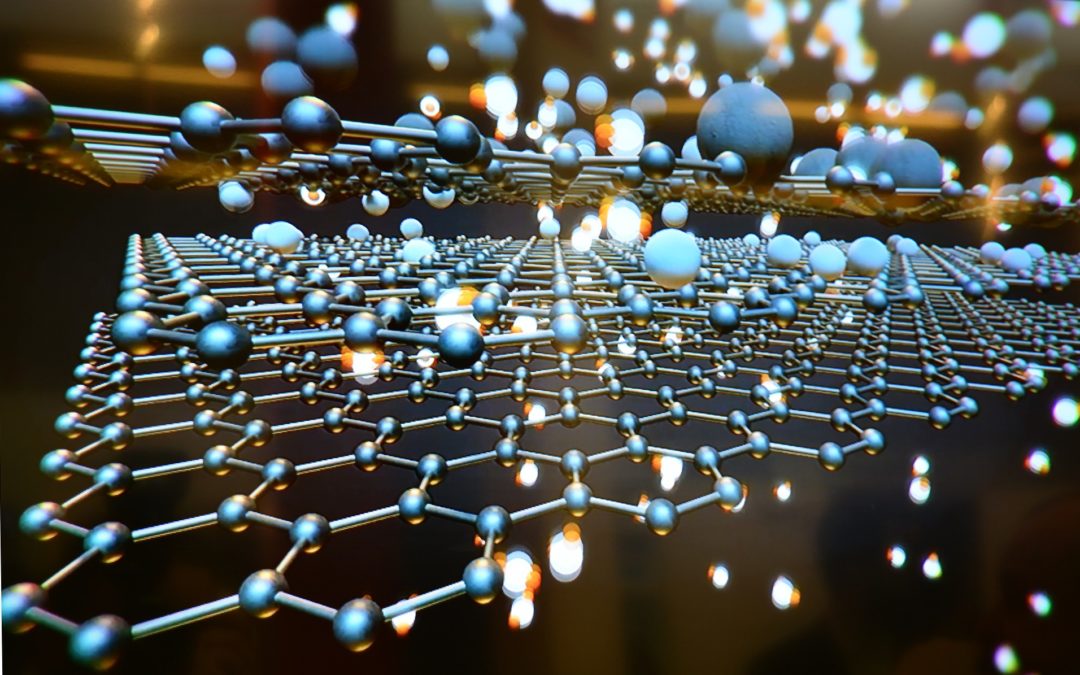
Graphene and superconductivity
How society is harnessing energy today is impressive, but scientists remain ambitious. They’re working to generate more power. A recent experiment with graphene sheets has led scientists to rethink our energy potential. Generating more energy, losing less of it and sending it longer distances give scientists a plethora of inventions to dream of.
What Graphene can Achieve in Superconductivity
In the form of what looks like an elastic sheet, graphene is made up of carbon molecules. It’s engineered as a flexible sheet that’s exactly one atom thick. Graphene is used as a conductor of electricity and heat. It strengthens other materials and can extend the life of LED light bulbs. Graphene was discovered in 2004 but has been steadily improved since. What’s stirring the scientific world up is the potential for graphene to be used as a superconductor.
This theory developed after two sheets of the carbon synthetic were found with a charge during a controlled test. One sheet sat at a 1.1-degree angle from the other. This resulted in the first known occurrence of independent power—as generated from graphene. Graphene sheets are constructed with hexagonal grids within them that create friction when moved against other sheets. That friction is now capable of generating 200 milli-electron volts.
Conducting Power with Greater Ease
Science would have a great deal to think about if we generated electricity at higher rates. The “twisted graphene discovery” produced electricity without compromising its momentum or charge. Movement is required for graphene sheets to conduct energy, and that’s why they’re twisted into each other. The mathematical degree of each twist must be just above absolute zero. The result is a superconductor that could generate electricity at greater speeds.
What Graphene Ultimately Means for Science
With the prospect of becoming a new superconductor, the graphene breakthrough created a fresh sense of potential within the scientific community. Though no applications of the technology have been put into patent form, thinking of what can be done is itself revolutionary. Society has a new way to define how electricity can be transported. Power like these options can even charge our vehicles.
What is a Superconductor?
A device that conducts electricity is a conductor. Superconductors, however, are designed to reduce friction and to increase the flow of electricity, heat or energy. Superconductors maintain their currents as an endless cycle of undisturbed frequencies. If you examined the state of the currents that you sent into a superconductor, you would find them energized at the same levels that they entered the enclosure with.
The world’s most famous superconductor puts the potential of graphene into a larger perspective. The Hadron Collider holds particles and electric charges. It’s designed to move currents and even atoms at the speed of light. The use of this particular superconductor requires high-powered magnets; it was built as a tunnel that’s 100 kilometers long.
Why are Superconductors Important?
Conducting power allows us to send, generate and ultimately use energy. Electromagnetic waves, as sustained by a superconductor, gives us the power to make x-rays of our bodies. This technology is used in hospitals and in MRIs. Some trains are powered by superconductors. The future of hoverboards might even rely on this type of power.
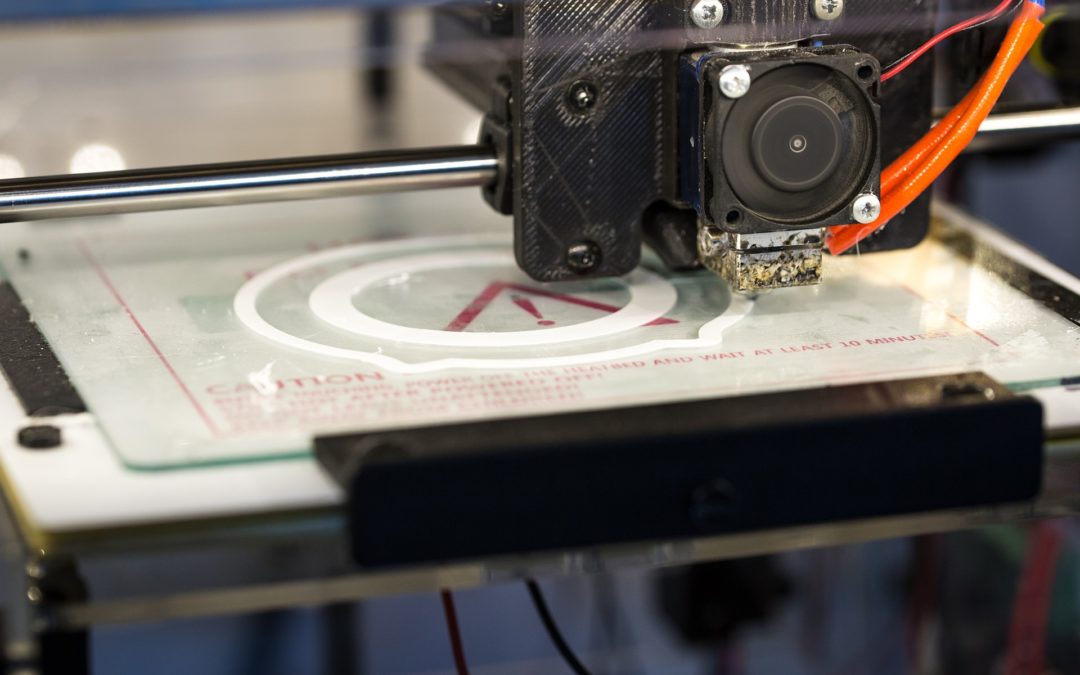
Graphene ink in 3D printing
Graphene and 3D printing represent two exciting developments in science and technology. Graphene has long been regarded as a wonder material. Made from carbon, it’s an extremely versatile material that has piqued researchers’ interest for years.
Graphene’s been seen as a wonder material because it has so many desirable characteristics. It’s good at conducting electricity. It’s a semimetal with lots of tensile strength. It lies flat, but at the molecular level its structure is similar to that of a carbon nanotube. The bonds that make graphene are neat, and there’s never any overlap between bonds. It’s a remarkably stable material, and lies very thin. In spite of all this promise, however, it’s been difficult for engineers and scientists to really unlock graphene’s potential. Until now, that is.
Recently, researchers in China combined graphene with 3D printing technology. By incorporating graphene into an ink that dries as a solid, they were able to print an object with highly conductive properties. By using a 3D printer, they were able to print three-dimensional graphene, a combination that the research team used to print supercapacitors. This technology could revolutionize the way energy is stored for electronics. These graphene oxide capacitors have the potential to reduce the size of batteries for a variety of electronic devices.
One unique thing about the process used by the research team is that they’ve devised a fairly green process. They used graphene sheets on a salt template, and made the deposit via chemical vapor. If this process can be scaled up effectively, it could change the way items like smartphone and laptop batteries are manufactured. However, at the present time, there’s not enough high-quality graphene that’s readily available to really scale this up.
Another issue is the form of graphene that’s used. In order to make the ink, powdered graphene is needed. In that powdered state, it’s hard to find graphene of high quality. Unusable graphene samples the research team encountered had a number of problems. Some had chemical impurities. Others didn’t have an even thickness. Still others had structural problems.
The technology used in the research team’s process helped to overcome many of these issues. To make the ink, graphene nanosheets were coated with nitrogen. The NaCl template used had advantages, too. Table salt is readily available. It’s water soluble, meaning it’s easy to remove from the ink after it’s in its final state. Originally created in a cage shape, the ink is collapsed into a 2D shape using ultrasound. At proper thickness, the graphene ink is just 5 to 7 graphite layers in thickness. When this composite ink was finished, it was easy to print flexible battery parts.
The researchers were then able to print flexible electrodes for supercapacitors. They were also used to print interlayers for Li−S batteries, along with other materials. In comparison to traditional batteries, these supercapacitors had improved conductivity. They performed better, and didn’t require the separators traditionally seen in batteries.
Because it’s affordable, lightweight and uses readily available materials, this technology holds great promise. It’s speculated that 3D printed graphene inks will have applications for wearables, phones and other smart devices. This method has the potential to make technology much more affordable to produce.
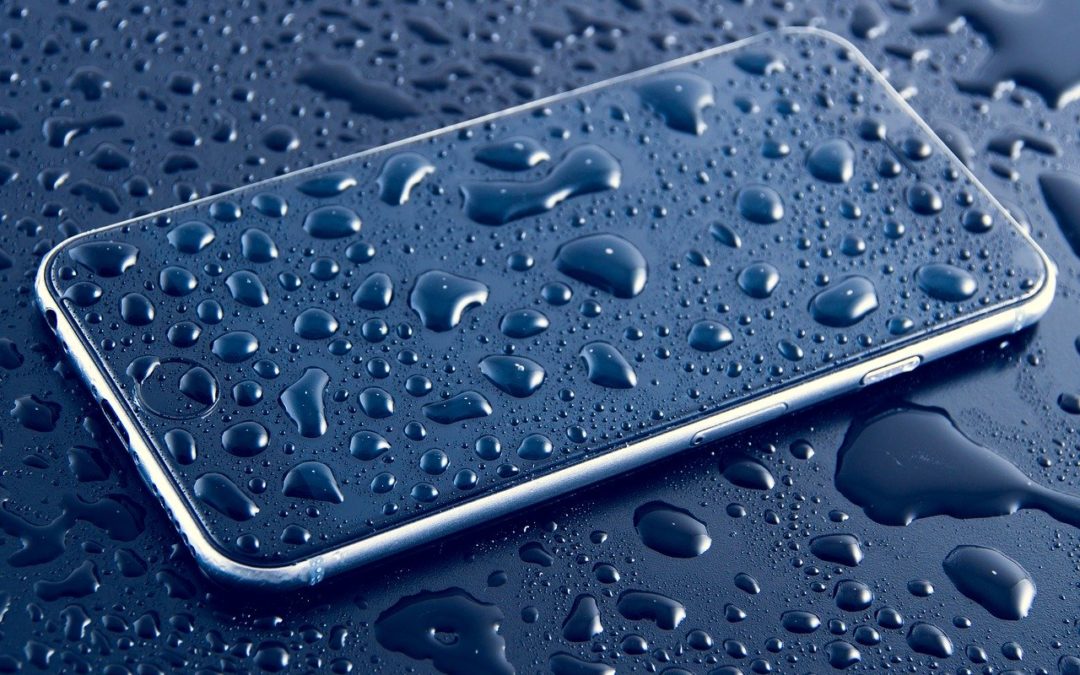
The complicated relationship between graphene and water
Carbon is one of the most common types of chemical elements found on earth. This versatile atom type can combine in a variety of shapes to form various materials, including graphene. Graphene is a new form of carbon developed in 2004, and it is notable for being a fine layer that is only a single atom thick. This unusually useful form of carbon is stronger than steel while being a great conductor of electricity and heat. One of the particularly interesting things about graphene is the way it interacts with water.
Graphene behaves somewhat strangely when it gets in touch with water. It is similar to graphite, a type of hydrophobic material. Graphene forms tight bonds that are so strong water cannot easily move through a sheet of graphene. However, since it is a very thin sheet that is only one atom wide in thickness, graphene normally breaks when it comes into contact with water. Another unusual fact about it is that the material also floats whenever it is placed on water. Due to all this behavior, scientists assumed graphene was a hydrophobic, or water-repelling, material.
It turns out that graphene is a heavily misunderstood material. A recent study from the Advanced Materials Journal has proven that graphene is actually hydrophilic. This revolutionary study was one of the first to manage to place droplets of water on graphene without the graphene immediately breaking up. Other researchers who have tried these tests supported graphene on metal or silicone which are hydrophobic. However, the Advanced Materials study used a thin layer of ice to support the graphene instead, so they could see how it truly interacts with water. When they did this, the researchers found that the water was actually attracted to the graphene sheet. This conclusively proves that graphene is a hydrophilic substance that attracts water to itself.
Now that researchers better understand graphene’s relationship to water, they have been able to develop a lot of extremely useful applications for graphene. Graphene is an unusually thin arrangement of carbon atoms, so it is suitable for a lot of modern scientific and engineering techniques. Since it can actually attract water, it may be particularly useful as a method of filtering water. There are already some water filters currently in place that use layers of graphene oxide to filter salt out of water quickly and easily. The technology is still fairly new, but many hope that it will revolutionize how water is filtered and make it far easier for people to cheaply create clean water.
As the Advanced Materials study shows, graphene’s hydrophilic properties are more easy to observe when it is layered between water. Therefore, researchers are starting to look into all sorts of constructions that involve graphene sandwiched between liquids. There is some hope that hydrophilic graphene can be useful for constructing certain biosensors, fuel cell membranes, and even bulletproof vests.
Water can pose a major problem for many materials. While graphene’s connection to liquid is initially vexing, its properties prove that it is capable of interacting with water in ways that allow for new innovations and technologies to arise.
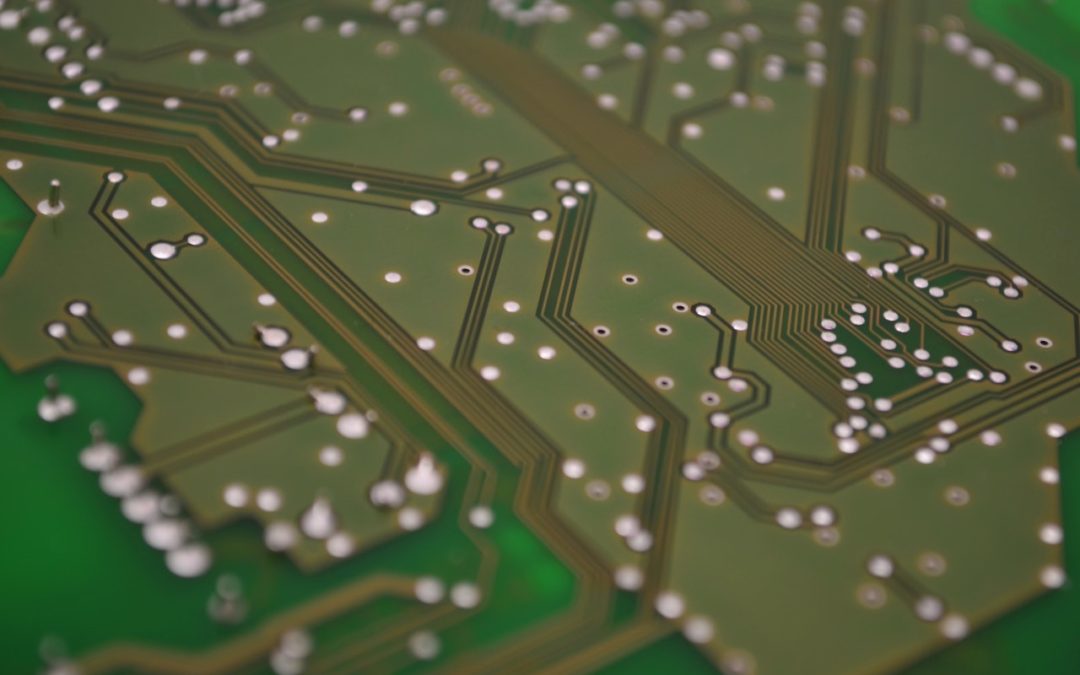
Is borophene graphene’s first true competitor?
At the bleeding edge of the tech wars, flexible atom-thick materials have been causing a stir among engineers since the late 1980s, when graphene was finally synthesized. Graphene is highly valued for its high conductivity, great flexibility and amazing strength. Research has shown great potential in printing graphene for paper-based electronic circuits, electronics applications, computer memory and improved vehicle batteries.
Around 100 times stronger than steel, graphene is almost transparent and a highly efficient conductor of both electricity and heat. However, because graphene is very rigid, there are hard limitations on flexible applications. Graphene can be difficult to stretch in other processes.
Beginning in 2014, researchers began to identify and finally to synthesize a new nanomaterial, borophene. An atom-thick sheet composed of 36 boron atoms in a triangular lattice, borophene forms hexagonal holes in the centers of the molecules. The result is a stronger, less expensive and lighter material with similar properties to graphene. Is there really any need for both nanomaterials?
Although graphene performs far better in touch screen designs than the currently standard indium-tin oxide, the big holdup is its sheer cost. A one-micron flake of graphene is currently valued at over 1,000 U.S. dollars. One of the most expensive substances on the planet, graphene is far too expensive to justify such a use in mass manufacturing.
Soon there were other nanomaterials with similar applications, including silicene, germanene, molybdenum disulfide, graphitic carbon-nitride and hexagonal boron-nitride, which led to the synthesization of borophene. Borophene’s properties are similar to those of graphene, but it’s much stronger, far more flexible and significantly lighter.
Borophene is the most flexible nanomaterial produced to date. Interestingly, the unique properties of boron at the molecular level mean that borophene actually becomes stronger when placed under strain, rather than shattering or cracking. Such properties offer the possibility of materials that are custom tuned to specific stress requirements.
With a higher electron density than graphene, supercooled borophene may be able to conduct electricity with no significant energy leakage. This could exponentially increase both the power and speed of computing electronics. Borophene might just make historic differences in computing power, with great promise for stabilizing quantum computer technology.
When borophene is accumulated on a silver surface from a vapor, it creates a rough surface with astounding levels of electron conduction along ridges in the surface. With directional structures like ridges that can be used as switches, borophene holds great promise for light polarization applications.
With exceedingly light weight, high strength and the ability to stretch while conducting electricity, wearable device technology would become far more practical, allowing embedded devices in clothing for life support reasons. Because boron has high chemical reactivity, sheets of borophene could easily be bonded to sheets of other nanomaterials to tune their properties for specific applications.
Graphene has created a market that has now reached over nine million dollars. Borophene is lighter, stronger, better conducting and possesses material properties that can’t be duplicated by graphene. Perhaps most important, borophene is far cheaper to manufacture than graphene, bringing some previously impossible ideas into the realm of likelihood. But borophene is not without its own disadvantages. With more research and time, it’s very likely that we’ll see both graphene and borophene fulfill a wide variety of roles in our society.
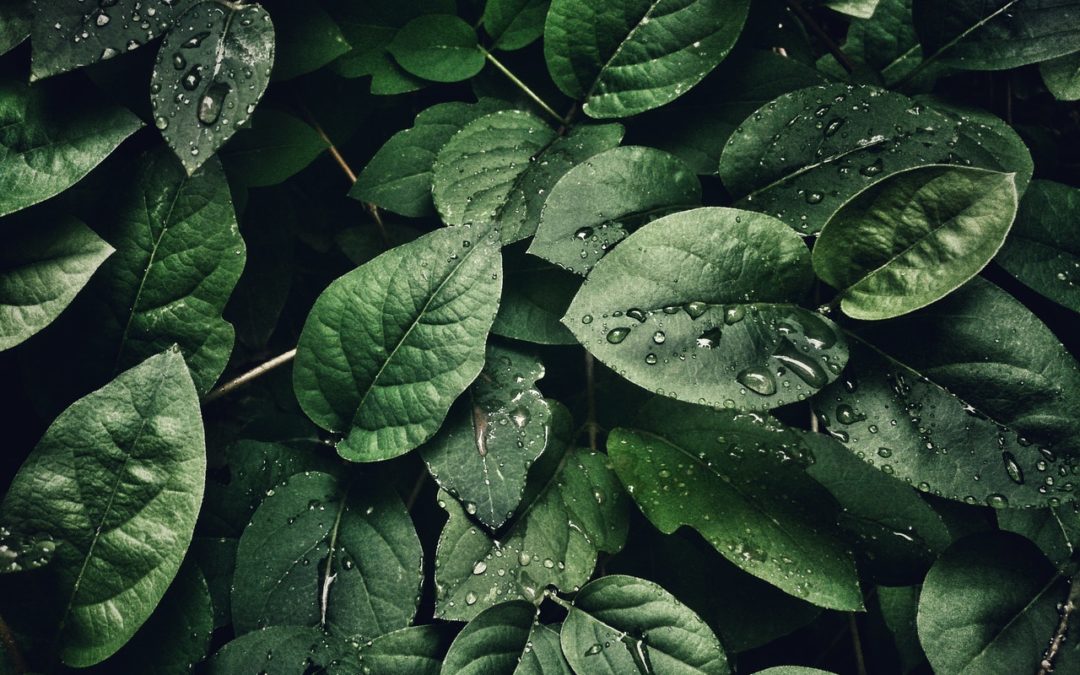
Can nanomaterials help create super-plants?
While science-fiction authors have long penned about hybrids between humans and machines, “super plants” that contain integrated nanomaterials might be much closer to science fact than cyborgs are. Today, scientists report that there are plants in development which can make nanomaterials; these are called metal-organic frameworks, or MOFs, and are applied as coatings on plants. These augmented plants might one day perform useful functions such as the sensing of chemicals or the more efficient harvesting of light.
Plants often endure blazing-hot long days to bear the vegetables and fruits their growers desire. The ultraviolet or UV rays of the sun can be intense; enough so to damage some crops. These plants might well thrive if they had a hand from some built-in sunscreen. A family of MOFs can absorb UV radiation that is harmful. Some MOFs can turn UV rays into other wavelengths, such as those that plants can utilize for photosynthesis.
A nano-engineer, Joseph Richardson, is working to lead a research team that seeks to make plants uptake the MOFs’ building blocks. Their goal is for plants to make their own MOFs. MOFs are too big to be taken up by plant roots. Cutting plants open to cram them full of nanoparticles would cause damage to the stems. So this team seeks another way. If these MOFs can capture UV rays that damage tissues, they might assist crops in surviving tougher climates still, both on Earth’s surface and out in space.
Richardson had a realization that the building blocks of MOFs are quite small; so small, in fact, that plant roots can drink them up. Now the building blocks must assemble within the plant and grow, on-site, to complete MOFs. The metal atoms and unique carbon compounds that comprise the building blocks were dissolved in water. Plant cuttings were then placed in this solution. It worked; these simple materials, absorbed by the plant, grew into fully-formed MOFs.
The scientists caused the MOFs in question to emit a green light that was intense when irradiated with the rays of UV light. This helped confirm that the plants were building MOFs. The entire plant fluoresced under the UV light. MOFs were formed in the stems, leaves, roots, and other plant parts.
Researchers are examining the protective qualities of the nanomaterials with promising preliminary data. The team tried coating clippings of lilyturf and chrysanthemum with luminescent MOFs; these were exposed to UVC light for several hours. After the three hours, compared with uncoated clippings, the MOF-ridden plants showed less bleaching and wilting.
MOF durability is a concern of C. Michael McGuirk. This materials chemist is worried that MOFs break down as time passes, losing their properties and unique structures. He mentions that this happens especially in water, which is a requirement of plant growth.
The next step is studying the MOFs’ effect on the growth of the plants they are taken up by. So far, toxicity has not been noticed in the nanomaterials. The MOFs may even someday help plants to grow better. This could lead to exciting new applications in agriculture. Hostile outposts, including space, may one day be fed by these MOF-embedded plants.
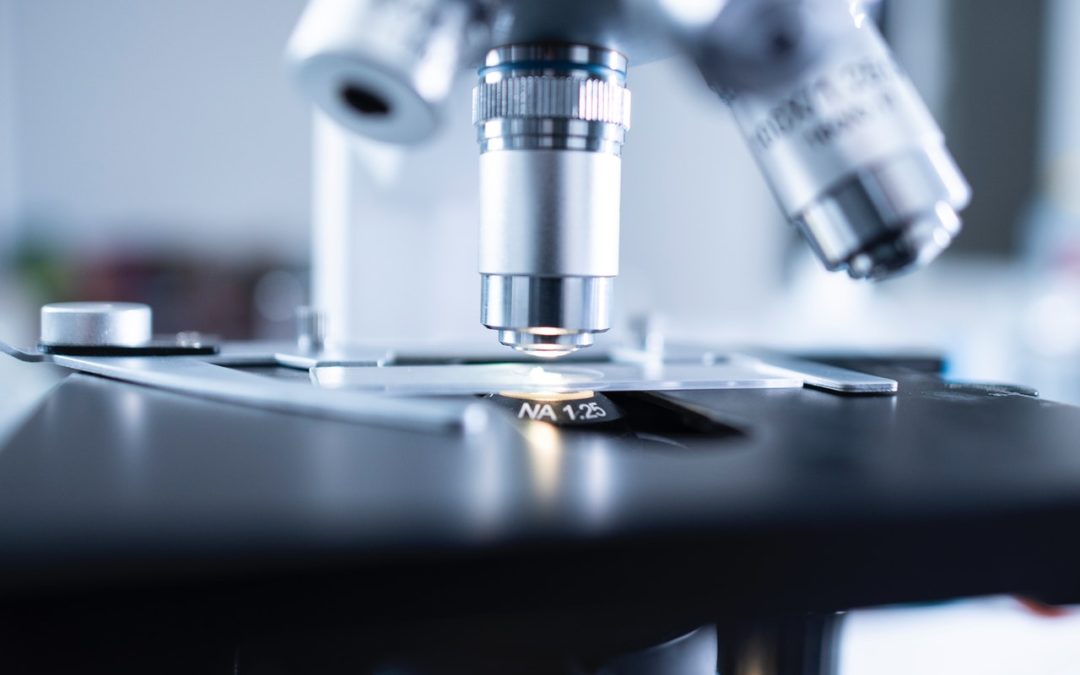
Graphene and resilient biotech
A team of researchers, chemists, and scientists recently created a new medical-grade biotech material that is being called a graphene hybrid. The material is made by combining standard graphene-based nanoparticles with hydroxyapatite (HAp). For anyone unfamiliar with hydroxyapatite, it is a ceramic derivative commonly used in medical and dental implants. Once graphene is combined with hydroxyapatite it forms to create a highly durable material that could potentially last longer than any biotech material currently on the market. The material is also completely non-toxic and it is safe for nearly all types of surgical applications, including open heart or brain surgery.
Problems With Non-Graphene Biotechnology
Over the last decade, the leading biotech implant material available is biometallic. Biometallic materials are commonly used in dental implants, stents, and even pacemakers. The downside to using biometallic materials is that in some cases it can corrode quite quickly, especially if it is heavily exposed to bodily fluids such as saliva, blood, or water. Once biometallic corrosion occurs it can leak particles into the surrounding tissues and into the bloodstream, causing inflammation, toxicity, and even death in high doses. Another major downside to biometallic materials is that they have a high thermal expansion rate which is known to cause nerve pain.
Ways Graphene Can Improve Biotechnology
It’s Non-Corrosive: One of the biggest benefits to using graphene in biotech materials is that it is non-corrosive, so there is zero risk of it ever causing toxicity, blood poisoning, or tissue inflammation. With zero risk of corroding, graphene can be used in medical applications where standard biometallic materials are prohibited. That means that graphene has a much broader application range. It could be used in artificial heart valves, dental implants, artery stents, and more.
It Has a Low Thermal Expansion Rate: Most biotech materials such as biometallic substances have an extremely high thermal expansion rate. What that means in layman’s terms is that biometallic materials will rapidly expand in size and temperature any time they are exposed to prolonged periods of high heat. Graphene, on the other hand, isn’t easily affected by hot temperatures that would be experienced within a human body. That means the risk of thermal expansion for graphene is very low.
Graphene is Non-Ferromagnetic: Some biometallic materials are ferromagnetic in their composition, meaning they create a magnetic reaction. Ferromagnetic materials used in biotech applications are less than ideal due to the fact that once these items are implanted into the body, the person receiving them cannot ever undergo a magnetic resonance imaging (MRI) scan. Graphene, on the other hand, is completely non-ferromagnetic, meaning it does not possess any magnetic quality.
It’s Cheaper to Produce: Another major advantage to using graphene materials in biotechnology is that it is substantially cheaper to produce than any other biotech material. In many cases, graphene is up to 100 times cheaper to produce. Biometallic materials, for example, are extremely expensive to produce due to the fact that the substances needed to make it require finite metallic resources. Graphene is like plastic in the sense that it does not rely on finite resources in order to be mass-produced.
It’s More Durable: Some early tests have found that graphene infused with hydroxyapatite creates an end material that could be up to 100 times stronger and more durable than the strongest non-graphene biotech materials currently on the market. What this implies is that the risk of a graphene biotech application malfunctioning is very low. It also indicates that most graphene-based biotech materials could be implanted into the human body for life, with no replacement ever needed.